
Yonatan Munk
On November 9, 2011, our special guest (via Skype) was PhD candidate Yonatan Munk, from the Department of Integrative Biology, University of California at Berkeley.
His class appearance can be seen in full on track 12 of the Bio-Aerial Locomotion collection on iTunes U.
Yonatan Munk is writing his dissertation at this point, after working on the gliding ants for about four years. His goal has been to determine how posture and morphology determine stability and control in gliding ants.
(To learn about what the canopy ants do, how they glide, and how they were discovered, read my blog post about Professor Steve Yanoviak, and his guest appearance in EK 131.)
When Yonatan started working on this problem, there was not much known about how the ants perform their gliding manoeuvres. Other gliding animals have obvious morphological adaptations for flight (e.g., a patagium), but ants do not have any obvious morphological structure that implies the functionality of a wing.
Yonatan has approached the problem by means of field work and dynamical simulations in the lab. One of the challenges relates to studying the shape of the ants' trajectories as they glide. Trying to measure three-dimensional trajectories in the field using synchronised cameras is possible, but as the ants fall they appear as only a couple of pixels in the digitally acquired video.
To be able to study how the ants use posture in order to influence their glide trajectory, Yonatan built a small wind tunnel that he could take to the field, where ants can be filmed gliding in a constant updraft. He has also done experiments in scaled physical models in the lab, using 3D-printed models that are towed in a tank full of mineral oil.
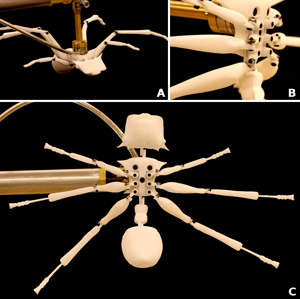
Physical model of the gliding ant used for dynamic experiment by Yonatan Munk.
One of the things that he found through his research is that leg length is very tightly correlated with gliding performance in the ants. Short-legged ants have terrible stability while gliding. But leg length is not an adaptation for gliding; long legs is a fairly common adaptation for ants that do a lot of foraging up on the trees (even many that don't glide). So this adaptation is being co-opted to help gliding performance. Gliding is really a behavioural adaptation to allow for a particular escape response from predators in the canopy environment. But gliding is still fairly uncommon, even in canopy specialists.
Here is a sample of some Q&As during Yonatan's guest appearance in the Bio-aerial Locomotion class.
- What is the reason for these ants gliding backwards?
It's actually quite difficult, based on the distribution of mass and aerodynamic forces, for the cephalotes to glide forward. They naturally generate a backwards force when their legs are placed in the skydiving position. And it's not really a problem for vision, in their case, because the cephalotes ants have their eyes set fairly wide on a wide head, so they have a clear line of vision behind them. It's certainly spectacular, as the first example ever seen of backwards gliding. But it turns out that it's a natural way for these ants to exert control.
- Is there any technology that these results can be applied to?
This whole idea of controllability is a really hot topic in the field of micro-air vehicles. This is the main technological application: developing small, autonomous flying robotic machines. We're a long way off from having something like that that works, or even closely approximates the performance that we see in insects. But people are making really significant progress in building small man-made machines that can fly. For controlling aerodynamic forces in these small robots, some measure of control outside of the wings is almost certainly going to play a part of it. Most insects use their legs and so on, especially in gusty conditions, do aid control. The findings that we are making with these ants may be relevant at some point.
- Are the ants able to figure out (with their tiny brains) where they are going, what their orientation is, where it wants to land and figure out how fast it's approaching a tree and latch on appropriately?
Those are really cool questions and they are all wide open at the moment. It would be great to try to get an idea whether gliding ants have more of their brain devoted to visual processing than a regular ant, for example. Remember that reproductive ants within the same species have wings, so just expressing a couple of genes differently results in an individual that can fly and has a brain devoted to flight. So it's not completely out of the question that the workers might have some of that processing ability as well.
- What will you work after you finish your dissertation?
For my postdoc at the University of Washington, I'll be working on moth flight. One of the things that I'm interested in as a result of all this work with the gliding ants is how widespread is this idea of leg use and abdomen use in flight control in insects that actually have wings and fly. So I'm going to look at how these appendages are used in moth flight for auxiliary control.
- Any ideas of things for engineering students to do?
There's a ton of unexplored territory. There are many insects that have not been studied at all, and their gliding performance has not been determined but we know they glide. For micro-air vehicles, biological studies will eventually tell us a great deal about, not only biology and ecology, but how to design new devices. The whole idea of what the insects are responding to, in terms of neural control. The type of experiments that have been done with fruit flies using artificial optical flow, for example.
The interview ended Yonatan answering students' general questions about graduate school, emphasizing the importance of undergraduate research (consistent with the advice given by Prof. Robert Full in his guest appearance in the class).
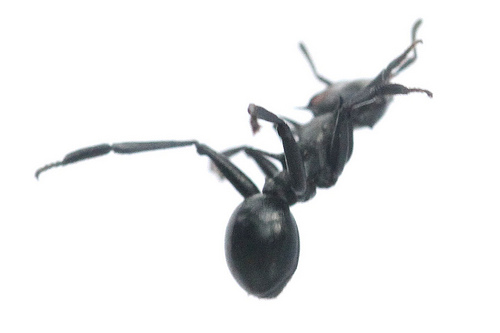
Canopy ant in gliding posture. Photo by Yonatan Munk.