The idea of shark attacks has terrorized humans for years, helped along by movies like Jaws. When we imagine them, we mostly think about the sharks’ vicious, powerful jaws, filled with hundreds of razor-sharp teeth that are so adept at killing. But what we don’t always think about is how they swim quickly, efficiently, and silently through the water towards their prey. What gives them this extra advantage?
Sharkskin isn’t smooth like many other fish, but instead made of many tiny, ridged scales called dermal denticles (pictured below). These ridges are arranged very close together and parallel to the shark’s direction of motion. While the dermal denticles have many functions, one of them is clearly to reduce a shark’s drag while swimming.
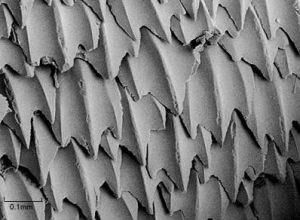
Close-up of the dermal denticles.
Drag, as we learned in class, is a force that acts on objects opposite to their direction of motion, when in a fluid like air or water. Engineers try to achieve laminar flow of fluid around an object, because then drag is often proportional to velocity (Fd ∝ v). But when flow becomes turbulent, Fd ∝ v2, so the drag force shoots up.
A shark without ridges would feel a turbulent flow of the water layer just above its skin, resulting in a large drag force. But with the ridges, the boundary layer of water is directed so there is little turbulence. It may seem strange that a rough surface is better suited for smooth swimming, but idea is not unheard of. For example, without their dimpled surfaces, golf balls would not be as aerodynamic.
Aircraft engineers have recently been looking to mimic the ridges of sharkskin. Airplanes are subject to a very large drag force, and 40% of that comes from turbulence on the boundary layer. Scientists at the Fraunhofer Institute in Germany have developed paint for aircrafts that is inspired by sharkskin. The paint is made out of nanoparticles, and when applied using a special stencil, it forms grooves on the surface of airplanes, reducing the drag force.
This innovation has large environmental impacts. Engineers estimated that the paint reduces skin friction by 4-7%. This means that much less fuel is used up opposing the force of drag. If every airplane in the world used this paint, this could mean saving 4.48 million metric tons of fuel per year – good news for anyone cringing at the current gas prices.
So, next time you watch Jaws, keep an eye out for those energy-saving denticles – not just the terrifying teeth.
References
Anitei, Stefan. “The Shark Coating – Softpedia.” Latest News – Softpedia.
Reals, Kerry. “Aircraft Engineers Turn to Biomimicry for Greener Designs.” Aviation News and Aviation Jobs from Flightglobal.
“‘Sharkskin’ Paint Could Make Planes, Ships More Fuel-efficient.” Greenbang – Smart Technology Analysts.
“Skin of the Teeth.” ReefQuest Centre for Shark Research Home. Web. 25 Sept. 2011.