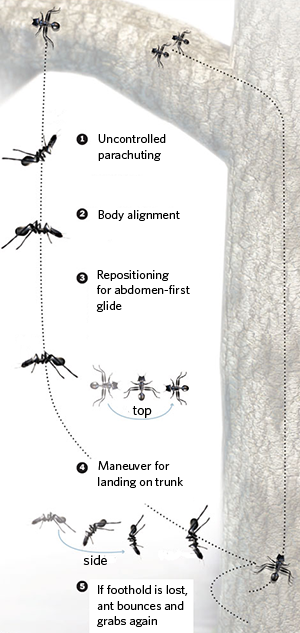
Graphic adapted from the New York Times article on the gliding ants. See references below.
Canopy ants use "directed aerial descent" to return to their home tree trunk when they fall from a branch. They were the first known case of gliding in an insect with no wings— a completely unexpected discovery. They also glide intentionally backwards toward the tree, with their abdomen (gaster) leading and their head tailing, which is a behavior never recorded before.
The whole maneuver forms a J-shaped trajectory, consisting of:
- a vertical drop (uncontrolled parachuting)
- quick body alignment, with the abdomen pointing to the tree
- a glide in a steep angle toward the tree trunk
Prof. Steve Yanoviak, at the University of Arkansas, who discovered the gliding behavior of ants, spoke to the class via Skype on Sept. 14, 2011.
A video of his guest appearance can be viewed, downloaded and sync'ed to your iPod visiting the course's iTunes U page.
This is an account of Prof. Yanoviak's Q&A session in "Bio-aerial Locomotion".
Answering the first question from a student, he told us that he was working in a project in Panama, spending a lot of time up on the trees. Up there looking down, he said, it's natural to think about how things fall. Between collecting data, he dropped some ants from the tree, and something didn't look quite right about the way they fell. For several years, this stayed in his mind, but he did not pursue the question. But about 5 years after that first observation, while in Peru in an unrelated project, he brushed off a bunch of ants from himself, and they all fell gliding back to the tree. That was the "wow" moment.
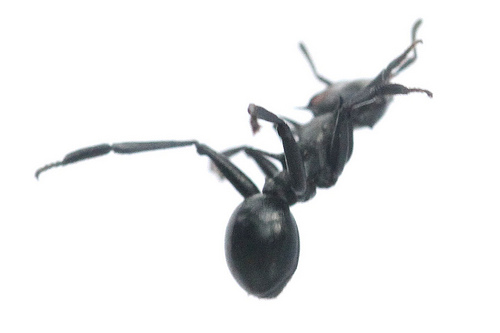
Canopy ant in gliding posture. Photo by Yonatan Munk.
Another student wonders why this behavior could not be related to the evolution of flight in other insects. Prof Yanoviak notes that ants are secondarily wingless, and they are very derived as a species. To understand how wings evolve, we have to look at insects that pre-date the evolution of wings, you have to go to a very primitive group. This is fortunately now the case with the follow-on work with bristletails.
What about the shape of the legs, which are shaped like a paddle? It turns out this is not relevant, since many other ants can glide, but they have cylindrical legs. However, the paddle-shaped hind legs make these ants better gliders. But they are also useful for other reasons in their environment: it enables them to avoid predation, or being trampled on by monkeys, and so on.
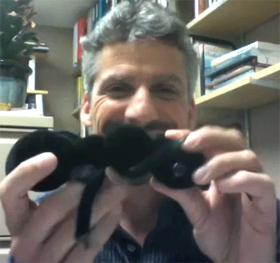
Prof Steve Yanoviak demonstrates the gliding maneuver using his stuffed toy ant
How do the legs help the glide? Using a toy ant, Prof. Yanoviak demonstrates how the ants fall from the canopy: they come in to the tree trunk rear-end first, and hold their hind legs and middle legs to the sky, similar to a skydiver. This puts the moment arms up above the center of gravity, allowing them to control better.
Is he still working on this topic? Although the big, burning, question has been answered, there are about a dozen other smaller questions that are waiting to be answered, but he currently does not have the time or the funding to pursue them. But he's working on some new projects, like the ecology of how ants interact with vines in the forest—ants preferentially use vines to climb in the forest, they use vines like their preferred highways, and he'd like to understand why they do that. The forest canopy limits the way ants can move to very limited path routes to access food. Vines are very efficient routes, and they can be defended from other insects.
How does he stay safe on top of trees? He uses regular climbing gear, throwing the rope around a big branch with a slingshot and a lead weight, and then strapping himself with a climbing harness; he is always tied to the tree as he climbs. If the tree is healthy, he is safe.
How much control do the ants have while they glide? "It's amazing," he says, and continues,
"You know, it's one of these things that, when I sit in my office and I think about it and I write about it, it's almost like 'hm, I really don't want to exaggerate this, is this really happening, as much as I think it is?'. And then I get out into the forest and I drop and ant, and it's just phenomenal. They make split-second decisions about their trajectory. They are falling very fast, between 4 and 5 m/s; that's fast fast, and I've seen them fall and they'll see, somewhere along that descent, they'll see a shiny spot, and you can see the ant change direction and start heading to that shiny spot, and at the very last second they realize, I'm assuming, they realize this is not the correct target and they change direction, and they'll find a new target, and it's only happening in a matter of a couple of seconds. It's really phenomenal."
And how do they figure out where they fell from the tree?
"It turns out, it doesn't really matter which tree trunk they land on. The density of trees in the tropical forest is so much that [...] if they go to the wrong target, they go to a different tree, as long as they climb up, they are very likely to find the pheromone trail of their sisters in the same colony. So all they have to do is get themselves back up on the canopy, and they are basically home free. Everything is so well connected up there, and these particular ants, they have very large colony sizes and very large foraging region, so if you fall out of the tree, especially if it's your nest tree, and you climb back up, you're almost certain to find either one of your nest mates, or a foraging trail of one of your nest mates."
And a final student question: Out of all the things that you've studied and researched, what have you found most interesting out of your results?
"You know, the gliding ant stuff, it really trumps everything else, I think. Most scientists, especially most field biologists, go through their entire career without making any kind of interesting discovery—by interesting I mean 'interesting to the public'; everything I do is interesting to me, I love my job, but the things that excite me maybe wouldn't excite you guys on a daily basis—so, there's nothing more rewarding than being able to make a discovery that people read in The New York Times and are going to get excited about, or that it's going to appear in Highlights magazine or Ranger Rick. To me, as a scientist, when the gliding ant story was in Ranger Rick, I felt like I'd come full circle, as a human being, you know, as a profesional; because, I remember sitting in the dentist's office as a kid, reading Ranger Rick, and getting excited about the little snippets about biology in there and reading them over and over again sometimes, and when the ants got in there, I thought 'oh yeah, that's it, this is more exciting than any other publication I've ever had.' "
References